Fusion energy, the power of stars, has long beckoned humanity. It represents an almost inexhaustible source of clean energy capable of addressing the energy challenges facing our planet. One of the most promising methods for harnessing fusion energy is through the use of tokamaks—devices that confine hot plasma using a toroidal magnetic field. However, on the path to economically viable fusion power plants, there are several significant obstacles to overcome.
The primary challenge lies in achieving a very high plasma density for the fusion reaction to proceed effectively. This density is limited by the so-called Greenwald limit—an empirical value above which the plasma becomes unstable. Achieving high density must be coupled with high-quality energy confinement, expressed by the parameter H98y2. Most future fusion reactor projects require simultaneously surpassing the Greenwald limit and achieving an H98y2 significantly greater than 1. However, until now, this task seemed unattainable.
Scientists from General Atomics (USA), in collaboration with colleagues from other countries, conducted a series of experiments on the DIII-D tokamak that could revolutionize our understanding of fusion energy capabilities. They employed a new operational mode called the high poloidal beta (βP) regime. In this mode, a special configuration of the magnetic field creates regions of enhanced density and temperature within the plasma, known as internal transport barriers.
The results were impressive. Scientists managed to achieve a plasma density 20% higher than the Greenwald limit and an H98y2 parameter 50% higher than the standard value for high-performance confinement regimes. This signifies the first time in the history of fusion research that parameters necessary for creating an economically viable reactor have been reached.
Furthermore, the new tokamak operational mode addressed another crucial issue—reducing the intensity of edge localized modes (ELMs). These instabilities lead to significant thermal loads on the reactor walls, which can cause damage. In the new operational mode, ELM intensity was significantly reduced, substantially extending the reactor’s lifespan.
Research showed that in the high βP regime, turbulent transport responsible for energy losses decreases at high plasma density. This is due to what is known as α-stabilization, which suppresses turbulence at high βP and local safety factor q values.
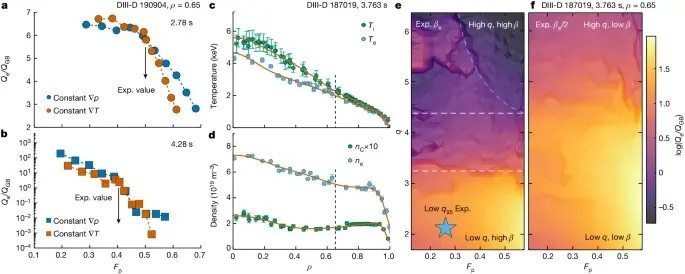
Thus, experiments on the DIII-D tokamak demonstrated that the high βP regime opens the door to creating a fusion reactor that meets requirements for density, energy confinement, and reduced heat loads on the walls.
Of course, there are still numerous challenges to overcome on the path to a fusion power plant, such as dealing with metallic walls and helium exhaust. However, the achieved results provide hope that fusion energy will become a reality in the near future.
If achieving high plasma density requires high deuterium density, why not use pure deuterium instead of a deuterium-tritium mixture?
Indeed, the deuterium-tritium (D-T) reaction requires high deuterium density. However, despite this, using pure deuterium is not the optimal solution. The D-T reaction has a much higher energy output and occurs at lower temperatures compared to the D-D reaction. This makes the D-T reaction more advantageous in terms of energy efficiency.
What are the main challenges that need to be addressed to create a fusion reactor based on the high βP regime?
Despite the promise of the high βP regime, there are still several challenges to be addressed:
Metallic wall operation: High-energy neutrons produced in the D-T reaction can damage reactor walls. Materials resistant to such effects need to be developed.
Helium exhaust: Helium, a byproduct of the D-T reaction, can accumulate in the plasma and degrade its parameters. Effective methods for helium exhaust from the reactor need to be developed.
Pulse duration: Currently, pulse duration in tokamaks is limited to a few seconds. To create an economically viable reactor, the high βP regime needs to be sustained for extended periods.
Can the high βP regime be used in existing tokamaks such as ITER?
Yes, the high βP regime can be implemented in existing tokamaks, including ITER. Research is currently underway to develop operational scenarios for ITER in the high βP regime.
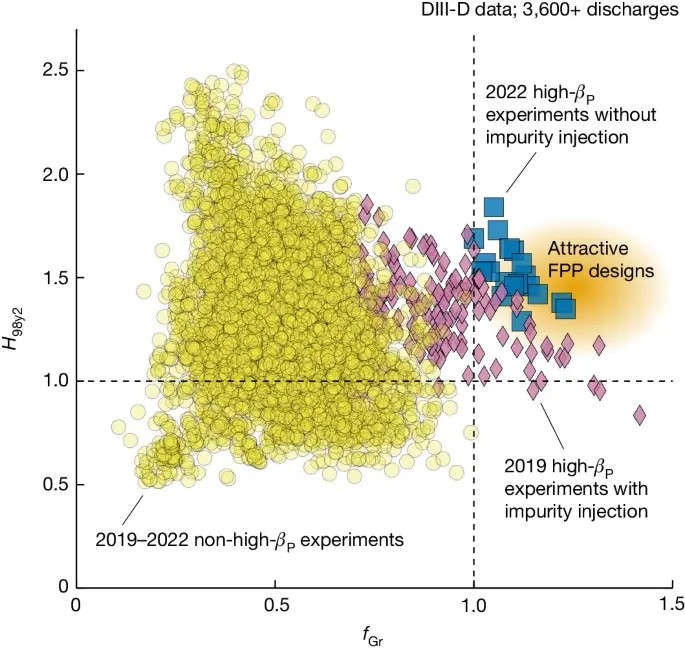